Is Nature Supersymmetric?
24 May 2011 | By
String Theory predicts a new symmetry, called "supersymmetry", that could shed light on some of today's mysteries of fundamental particles and interactions. In supersymmetry, every particle-type should have a "shadow" particle called a super-partner that (in general) has a much higher mass. The ATLAS Experiment has analyzed the first year of its LHC data and searched for evidence of these super-partners of ordinary matter.
In the proton collisions of the LHC, new heavy particles (the super-partners) could be produced. These super-partner particles would subsequently decay in a variety of ways, such as shown in Fig. 1, leaving many different telltale signals that ATLAS has sought to detect. Collision events with a so-called "momentum imbalance" are the key signature for the production of super-partner particles.
According to the law of momentum conservation, the momentum of all particles produced in the collision perpendicular to the proton-proton axis should exactly balance. An imbalance of the momentum would point to "missing" particles — particles that interact extremely weakly with matter. This imbalance occurs because the final decay products include particles that leave the detector without being detected (because they interact extremely weakly with matter). For the case of supersymmetry, these particles are the lightest super-partner particles (the "neutralino" ).
Since the neutralino does not decay at all, it is a permanent component of our universe. This particle might be the so-called dark matter that is 80% of all matter in the Universe. Therefore, these searches could shed light on the nature of dark matter.
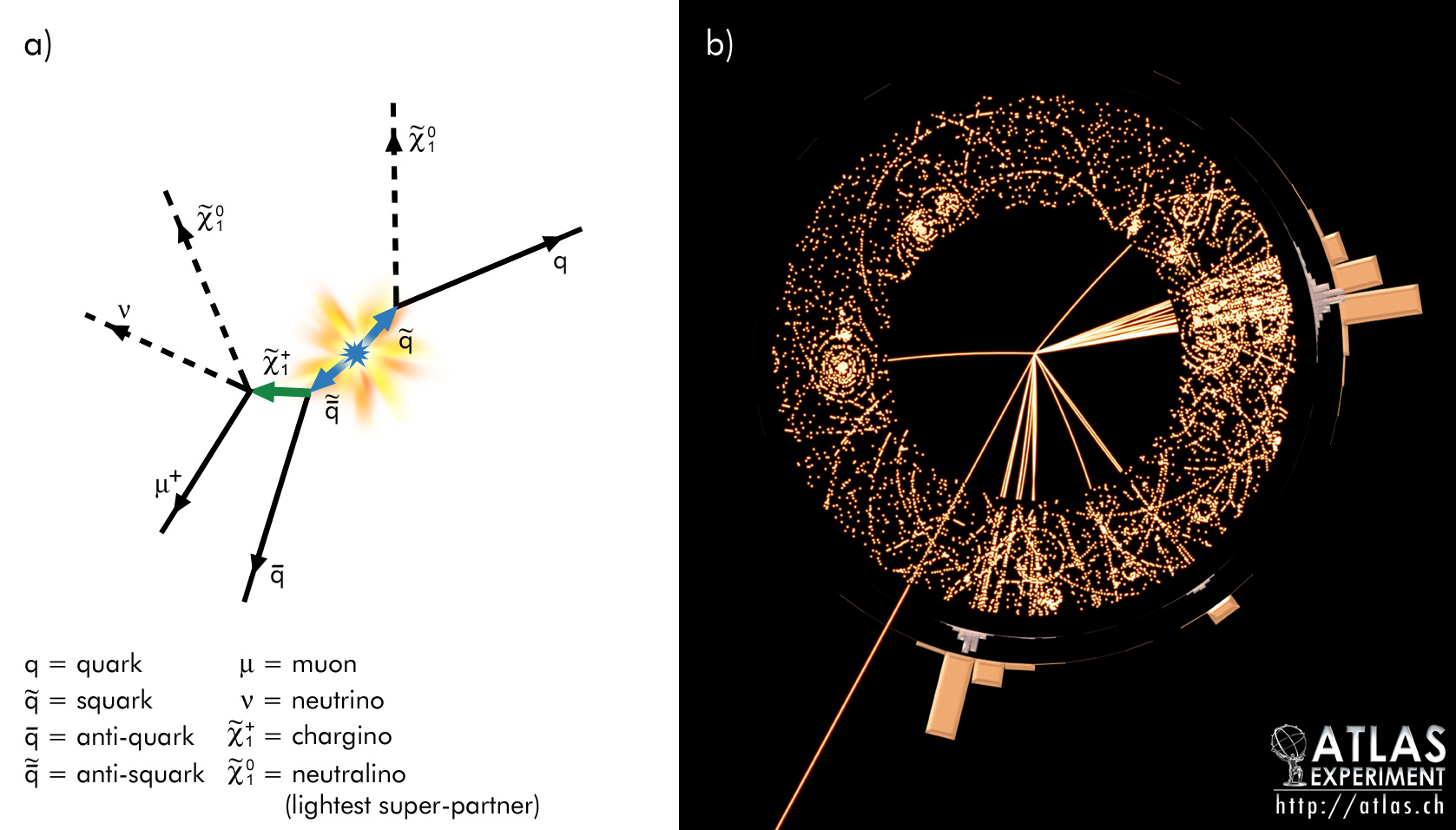
The measurement of the momentum balance requires the precise reconstruction of all types of measurable particles and a combination of the many component devices of ATLAS. This makes it one of the most challenging measurements at ATLAS.
In spite of many search attempts at colliders of lower energy, no evidence was found for the existence of supersymmetry. Why is that? It may be that the masses of the super-partner particles are so large that there has not been sufficient collision energy to produce them. They might only have been produced at the beginning of time, at the Big Bang. But with the high-energy collisions at LHC, this could change. Indeed, in order to explain the dark matter puzzle and other theoretical concerns, some of the super-partner particles are expected to have masses light enough to be produced at the LHC and be detectable in ATLAS, if they exist.
The ATLAS collaboration has now published the results of their first searches for super-partner particles based on the 2010 data-taking with proton collisions of energy 7 TeV. ATLAS has performed searches for super-partner particles giving rise to various decay products, including events with momentum imbalance plus 0, 1, or 2 leptons (electrons and muons). Up to now, there is no evidence for super-partner particles in ATLAS, and some mass regions have been excluded.
The excluded mass regions are usually described in terms of two mass parameters — which can be taken as the squark and gluino masses (the super-partners of the quarks and the gluons). For one of the possible supersymmetric models, the recent ATLAS results exclude the mass region shown in Fig. 2.
The mass limits correspond to the super-partner particles of the Standard Model quarks and gluons having a mass exceeding 870 GeV/c2 (corresponding to about 930 proton masses). This is shown as the point with R=1 in the figure. These results are presently the most stringent bounds on the mass of super-partner particles and a huge improvement compared to earlier investigations.
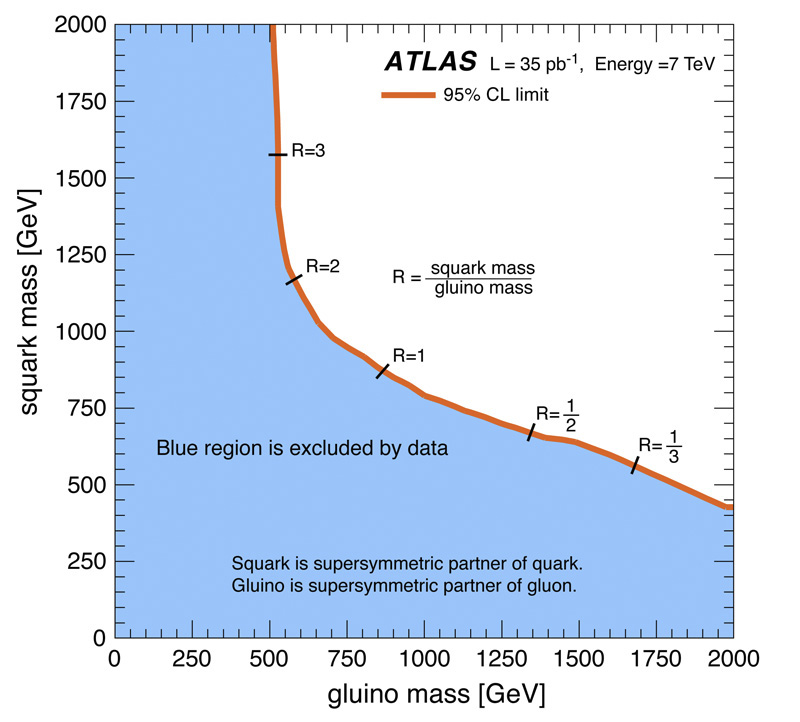
The Road to Discovery
The ATLAS results show that the masses of the super-partner particles are much larger than those of the ordinary particles. Because of these high masses, the super-partner particles are expected to be produced very rarely (the higher the mass, the more rare the production). Therefore, to achieve a reach to even higher mass ranges, ATLAS will have to continue accumulating high-energy particle collisions (events) to increase the chance of observing the super-partner particles.
On the road to discovery, one will first explore some of the lower mass regions, where a super-partner particle would be relatively easy to observe. At some point, events will start to show up that could be due to the production of super-partner particles — glimpses of interesting events will start to appear. But in the beginning, the number of event candidates might not be sufficient to claim a discovery, since some or possibly all of these events could also be due to processes other than the production of super-partner particles. To be able to claim a discovery, a large number of events will be needed, and several different analyses will have to make observations supporting the supersymmetry interpretation. This could happen soon, but it could also take time.