Searching for Electroweak SUSY: not because it is easy, but because it is hard
20 May 2019 | By
The Standard Model is a remarkably successful but incomplete theory. Supersymmetry (SUSY) offers an elegant solution to the Standard Model’s limitations, extending it to give each particle a heavy “superpartner” with different “spin” properties (an important “quantum number”, distinguishing matter particles from force particles and the Higgs boson). For example, “sleptons” are the spin 0 superpartners of spin 1/2 electrons, muons and tau leptons, while “charginos” and “neutralinos” are the spin 1/2 counterparts of the spin 0 Higgs bosons (SUSY postulates a total of five Higgs bosons) and spin 1 gauge bosons.
If these superpartners exist and are not too massive, they will be produced at CERN's Large Hadron Collider (LHC) and could be hiding in data collected by the ATLAS detector. However, unlike most processes at the LHC, which are governed by strong force interactions, these superpartners would be created through the much weaker electroweak interaction, thus lowering their production rates. Further, most of these new SUSY particles are expected to be unstable. Physicists can only search for them by tracing their decay products – typically into a known Standard Model particle and a “lightest supersymmetric particle” (LSP), which could be stable and non-interacting, thus forming a natural dark matter candidate.
If sleptons, charginos and neutralinos exist, they will be produced at the LHC and could be hiding in Run 2 data. New searches from the ATLAS Collaboration look for these particles around unexplored corners.
Today, at the Large Hadron Collider Physics (LHCP) conference in Puebla, Mexico, and at the SUSY2019 conference in Corpus Christi, USA, the ATLAS Collaboration presented numerous new searches for SUSY based on the full Run-2 dataset (taken between 2015 and 2018), including two particularly challenging searches for electroweak SUSY. Both searches target particles that are produced at extremely low rates at the LHC, and decay into Standard Model particles that are themselves difficult to reconstruct. The large amount of data successfully collected by ATLAS in Run 2 provides a unique opportunity to explore these scenarios with new analysis techniques.
Search for the “stau”
Collider and astroparticle physics experiments have set limits on the mass of various SUSY particles. However, one important superpartner – the tau slepton, known as the “stau” – has yet to be searched for beyond the exclusion limit of around 90 GeV found at the LHC's predecessor at CERN, the Large Electron-Positron collider (LEP). A light stau, if it exists, could play a role in neutralino co-annihilation, moderating the amount of dark matter in the visible universe, which otherwise would be too abundant to explain astrophysical measurements.
The search for a light stau is experimentally challenging due to its extremely low production rate in LHC proton-proton collisions, requiring advanced techniques to reconstruct the Standard Model tau leptons it can decay into. In fact, during Run 1, only a narrow parameter region around a stau mass of 109 GeV and a massless lightest neutralino could be excluded by LHC experiments.
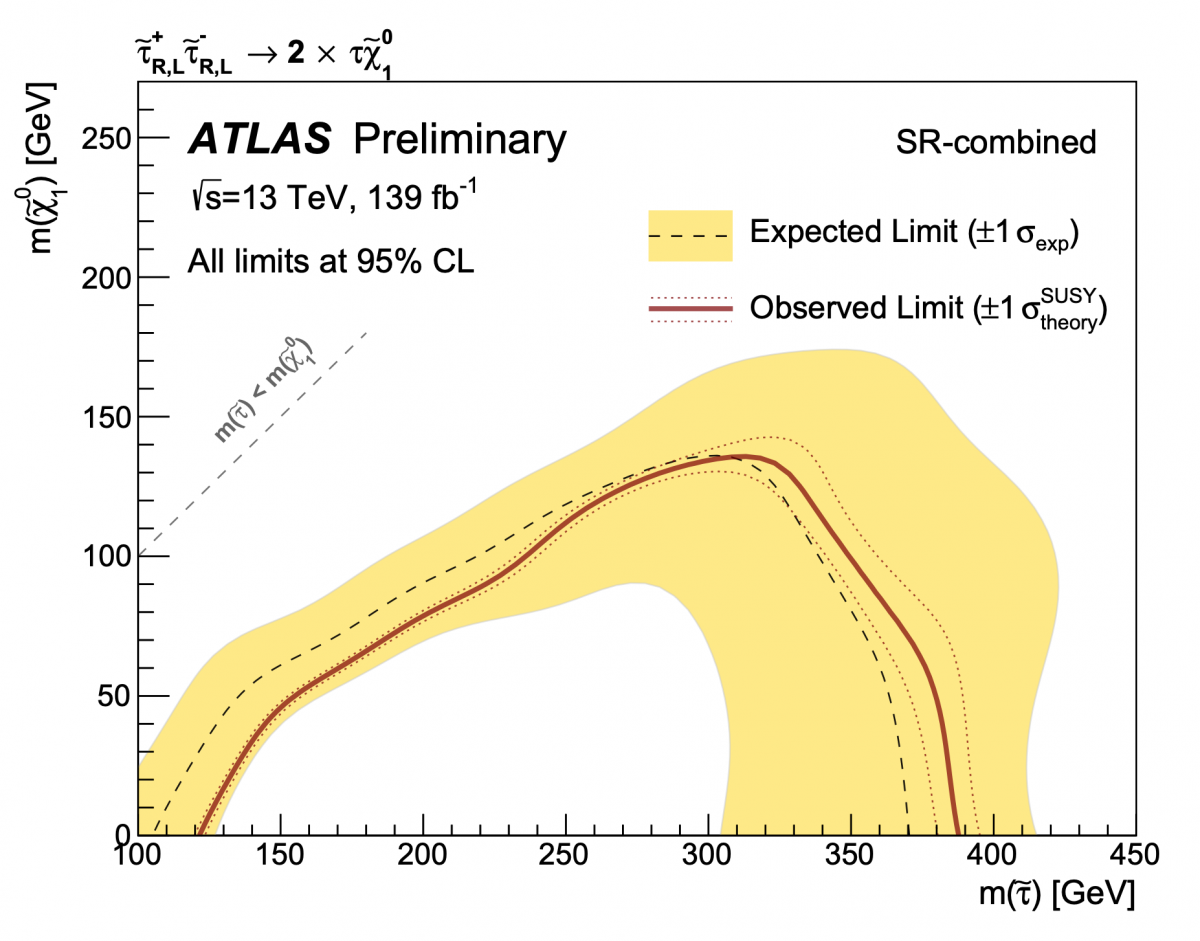
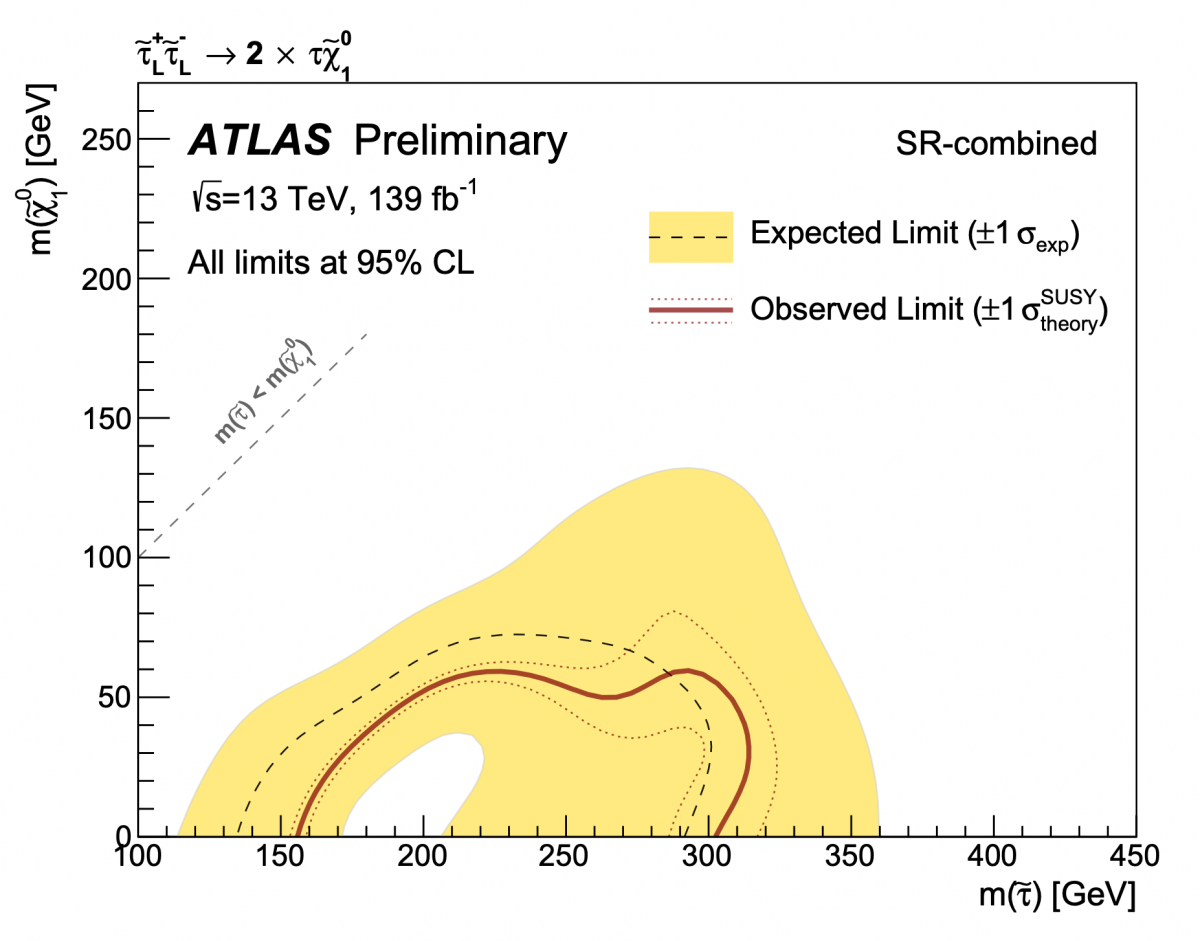
This first ATLAS Run 2 stau search targets the direct production of a pair of staus, each decaying into one tau lepton and one invisible LSP. Each tau lepton further decays into hadrons and an invisible neutrino. Signal events would thus be characterised by the presence of two sets of close-by hadrons and large missing transverse energy (ETmiss) originating from the invisible LSP and neutrinos. Events are further categorized into regions with medium and high ETmiss, to examine different stau mass scenarios.
The ATLAS data did not reveal hints for stau pair production and thus new exclusion limits were set on the mass of staus. These limits are shown in Figures 1 and 2 using different assumptions on the presence of both possible stau types (left and right, referring to the two different spin states of the tau partner lepton). The limits are the strongest obtained so far in these scenarios.
Compressed search
One of the reasons physicists have yet to see charginos and neutralinos may be because their masses are “compressed”. In other words, they are very close to the mass of the LSP. This is expected in scenarios where these particles are "higgsinos", the superpartners of the Higgs bosons.
Compressed higgsinos decay to pairs of electrons or muons with very low momenta. It is challenging to identify and reconstruct these particles in an environment with more than a billion high-energy collisions every second and a detector designed to measure high-energy particles – like trying to locate a whispering person in a very crowded and noisy room.
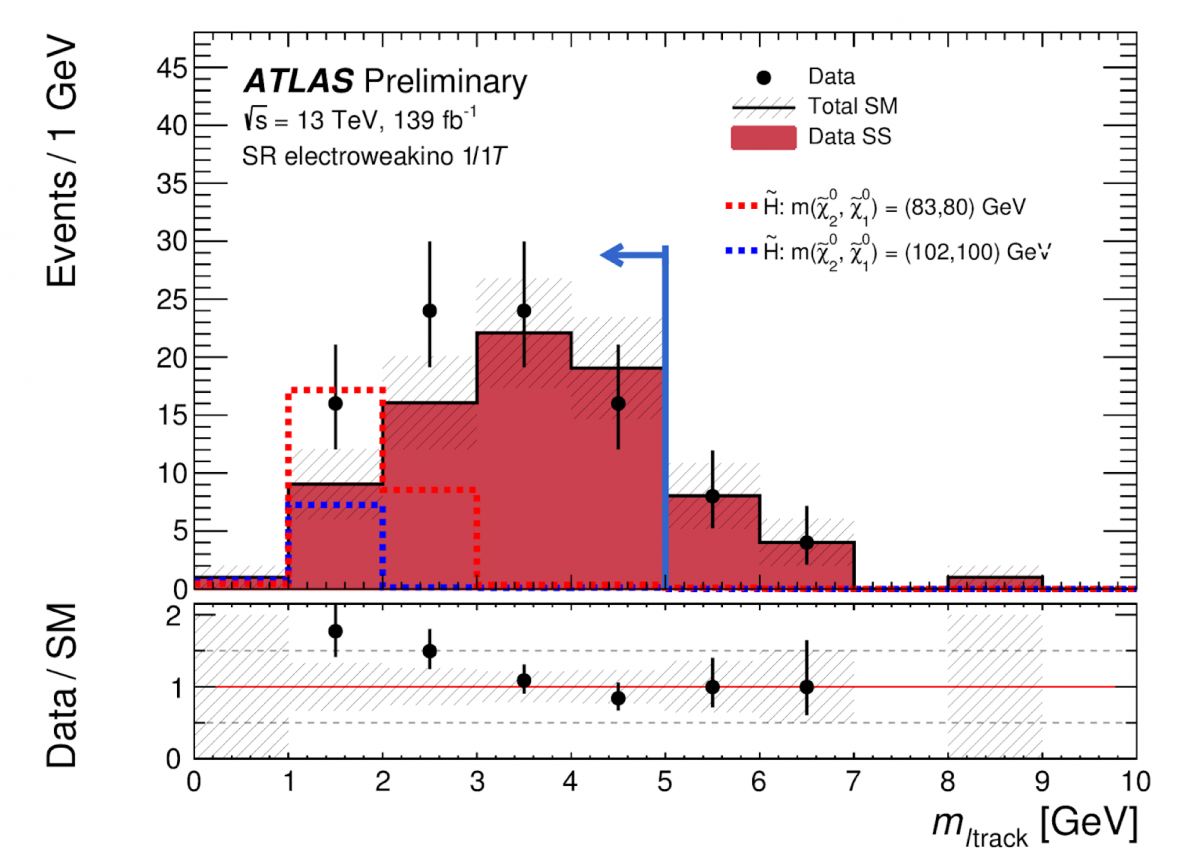
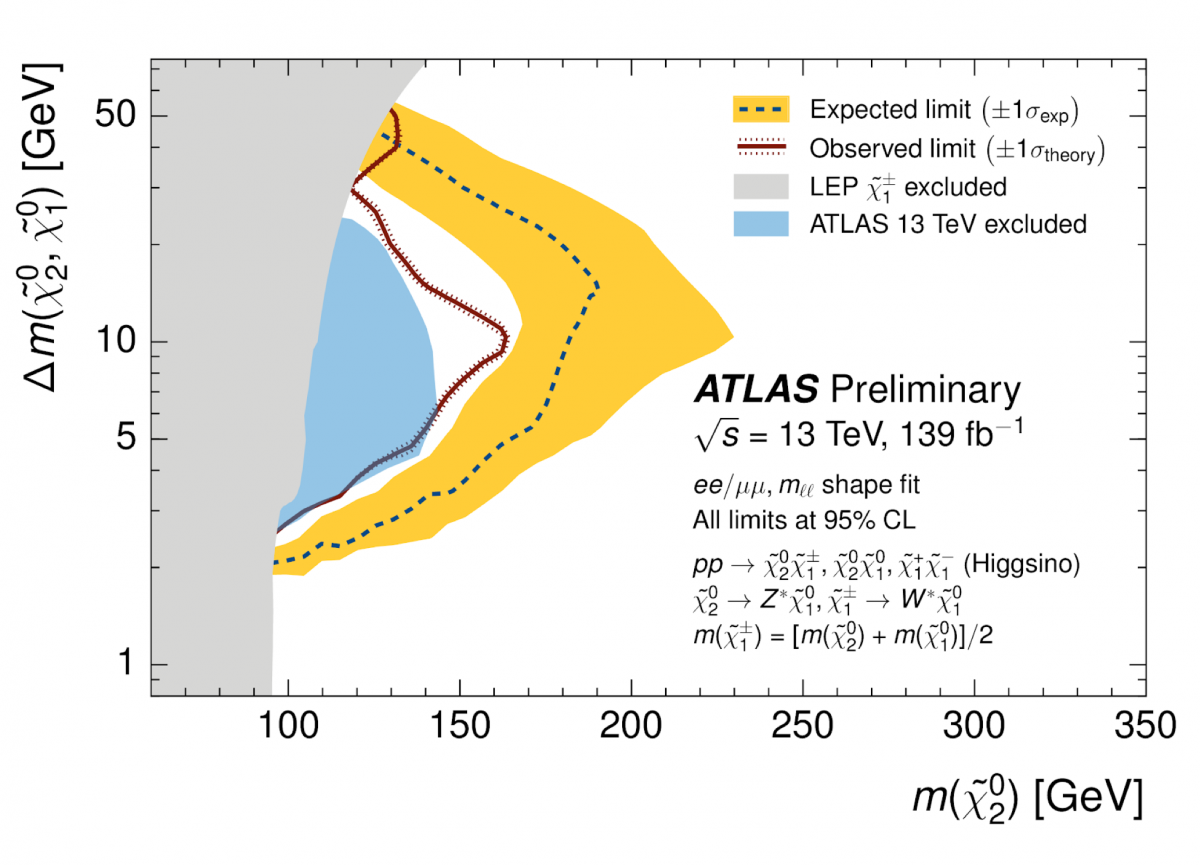
A new search for higgsinos utilizes muons measured with unprecedentedly low – for ATLAS, so far – momenta. It also benefits from new and unique analysis techniques that allow physicists to look for higgsinos in areas that were previously inaccessible. For example, the search uses charged particle tracks, which can be reconstructed with very low momentum, as a proxy for one of the electrons or muons in the decay pair. Because of the small mass difference between the higgsinos, the mass of the electron/muon and track pair is also expected to be small, as shown in Figure 3.
Once again, no signs of higgsinos were found in this search. As shown in Figure 4, the results were used to extend constraints on higgsino masses set by ATLAS in 2017 and by the LEP experiments in 2004.
Overall, both sets of results place strong constraints on important supersymmetric scenarios, which will guide future ATLAS searches. Further, they provide examples of how advanced reconstruction techniques can help improve the sensitivity of new physics searches.
Links
ATLAS presentations at the LHCP19 and SUSY19 conferences:
- LHCP19 plenary presentations: ATLAS Physics Highlights for LHCP 2019 by Kevin Einsweiler, Strong SUSY production by Bertrand Martin Dit Latour, and Electroweak SUSY production by Stefano Zambito.
- SUSY19 plenary presentation by Giordon Stark: SUSY in the ATLAS Experiment
- LHCP19 parallel presentations: Searches for stop and sbottom in ATLAS and interpretation to DM and LQ by Rosa Simoniello, Searches for staus by Alexander Mann, Searches for charginos and neutralinos in ATLAS by Luigi Longo.
- SUSY19 parallel presentations: Searches for promptly decaying squarks and gluinos with ATLAS by Julien Maurer, Searches for direct pair production of third generation squarks with the ATLAS detector by John Anders, Searches for charginos and neutralinos with the ATLAS detector by David W. Miller, Searches for sleptons with the ATLAS detector by Sonia Carra, Reconstruction techniques in supersymmetry searches in the ATLAS experiment by Christophe Clement, and Analysis description languages for LHC BSM searches by Sezen Sekmen.
New ATLAS results:
- Search for direct stau production in events with two hadronic tau leptons in 13 TeV proton-proton collisions with the ATLAS detector (ATLAS-CONF-2019-018)
- Searches for electroweak production of supersymmetric particles with compressed mass spectra in 13 TeV proton-proton collisions with the ATLAS detector (ATLAS-CONF-2019-014)
- Search for chargino-neutralino production with mass splittings near the electroweak scale in three-lepton final states in 13 TeV proton-proton collisions with the ATLAS detector (ATLAS-CONF-2019-020)
- Search for direct production of electroweakinos in final states with missing transverse energy and a Higgs boson decaying into photons in proton-proton collisions at 13 TeV with the ATLAS detector (ATLAS-CONF-2019-019)
- Search for squarks and gluinos in final states with same-sign leptons and jets using 139 fb−1 of data collected with the ATLAS detector (ATLAS-CONF-2019-015)
- Search for top squarks in events with a Z boson using 139 fb−1 of proton-proton collision data at 13 TeV with the ATLAS detector (ATLAS-CONF-2019-016)
- Search for direct top squark pair production in the 3-body decay mode with one lepton, jets, and missing transverse momentum final state in 13 TeV proton-proton collision data with the ATLAS detector (ATLAS-CONF-2019-017)
- See also the full lists of ATLAS Conference Notes and ATLAS Physics Papers.