The Higgs boson’s shadow
14 July 2014 | By
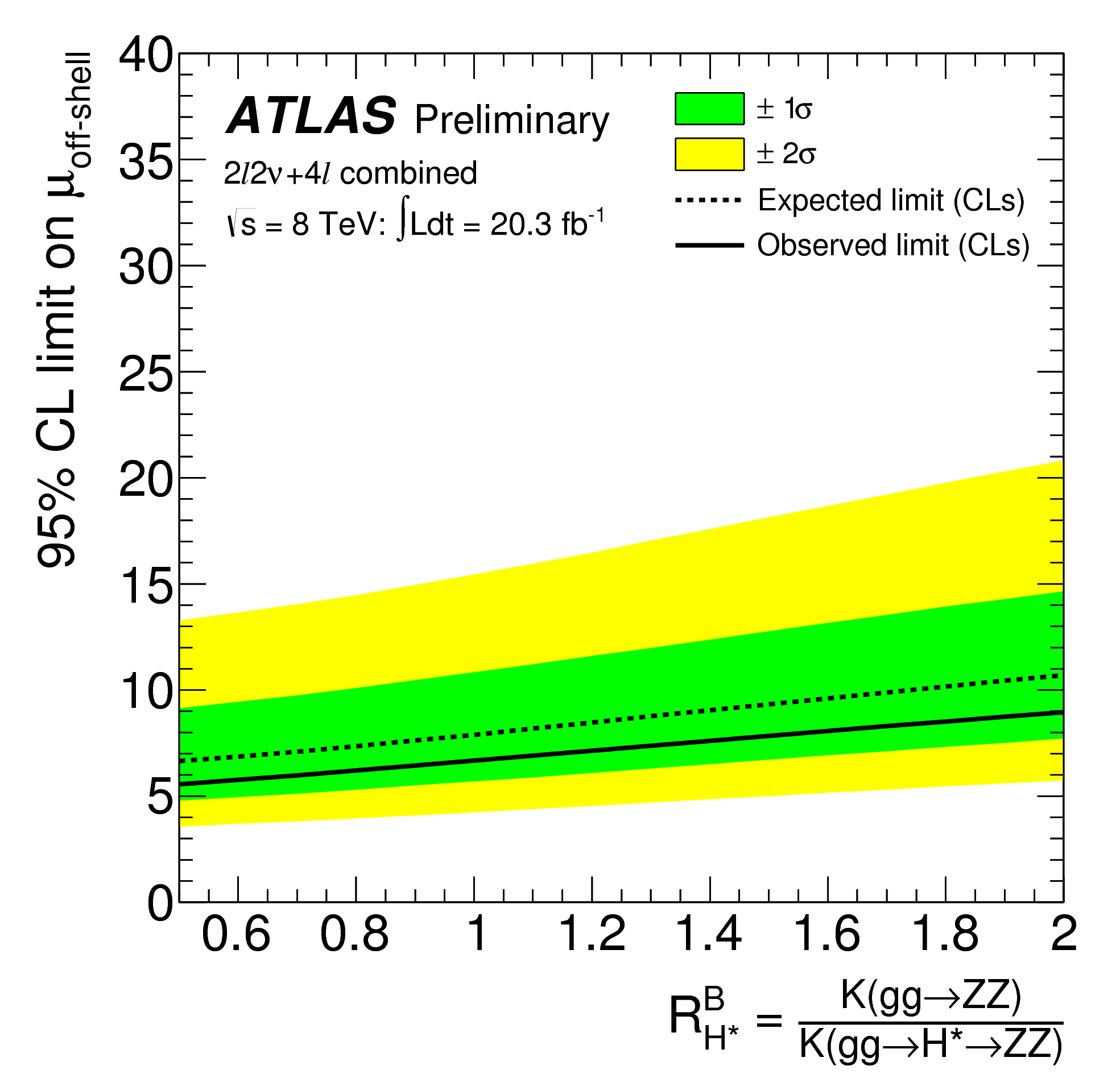
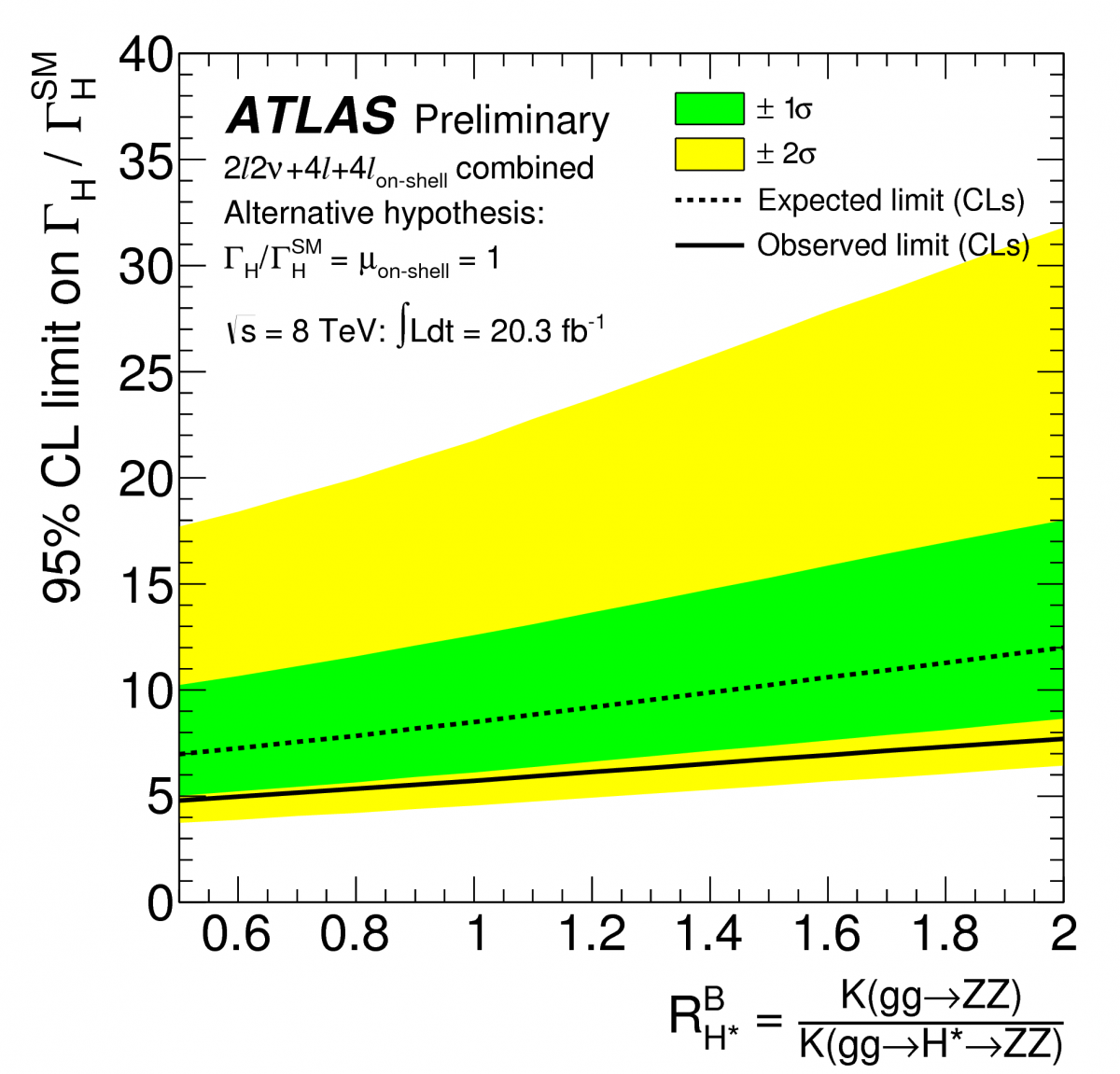
ATLAS physicists have studied the “shadow” of the Higgs boson far above its mass peak in an analysis of the full sample of 8 TeV proton-proton collisions delivered by the LHC in 2012. The study involves Higgs boson decays into two Z bosons, which themselves decay into four charged leptons or two charged leptons plus two neutrinos. Among other interesting properties, it provides new insight into the lifetime, or natural width, of the Higgs boson.
The Higgs boson was discovered by the ATLAS and CMS experiments in 2012 at a mass of 125 GeV. Ever since, the new boson has been under intense investigation. New analyses have been motivated by recent theory papers that predict contributions from the Higgs boson signal to high-mass event rates involving two Z bosons through what is called an “off-shell” component. Additional motivation comes from the possibility that new physics could alter how the Higgs boson interacts with other fundamental particles in this high-mass region.
The lifetime of a particle is governed by the reciprocal of its rate of decay (or its “decay width”). A large decay rate leads to a short lifetime. Although the Higgs boson decays fast - its mean lifetime is predicted to be only 1.6 10-22 seconds - it is not fast enough to make the Higgs peak broad. Its natural width is only 4.2 MeV, which is narrow compared to its mass, or even compared to the measurement resolution of the ATLAS detector. In spite of this small width, quantum mechanics allows in rare occurrences a Higgs boson of much larger mass (“off-shell"), which can interfere with other processes, not involving the Higgs boson. More abundant background processes, which effectively submerge these quantum phenomena, cannot be distinguished experimentally from the signal, and must be predicted by Standard Model theory.
An interesting feature of this analysis is that, while the amount of Higgs-boson signal observed on the 125 GeV peak (“on-shell”) depends on the Higgs-boson width, the far off-shell signal is independent of it. A comparison between the on-shell and off-shell rates therefore allows, under specific model assumptions, to extract information on the Higgs-boson width.
A proper estimate of the uncertainty in the prediction of the high-mass backgrounds is an essential ingredient for this analysis. The background process of two gluons producing two Z bosons, which is most similar to the Higgs signal and which interferes destructively with the signal, is known least precisely. Estimates indicate similar QCD corrections of a higher order for the signal – two gluons producing a Higgs boson which decays to two Z bosons – and background processes, but have not yet been calculated precisely. Therefore, the results are presented as a function of the unknown ratio between these two enhancement factors (Figure 1 and 2).
The sensitivity to the expected off-shell Higgs boson signal yield at high mass could not yet be reached as the measurement is limited by the small number of Higgs-boson events observed. Within the range shown, the off-shell signal contribution is measured to be lower than 5.6 to 9.0 times the Standard Model prediction (Figure 1). Assuming that the couplings of the Higgs boson are independent of the energy scale at which it is produced, this off-shell measurement can be combined with the on-shell Higgs signal around 125 GeV. A limit on its natural width, which is connected to the lifetime of a particle, is set between 4.8-7.7 times the predicted Standard Model width (Figure 2).
This analysis follows a similar result reported earlier this year by CMS and, until recently, it was assumed that this level of precision was not achievable at a proton-proton collider. The technique provides a unique method for probing a key property of the Higgs boson and the precision of the measurement is expected to improve in the coming years with the increased statistics to be provided by the LHC.